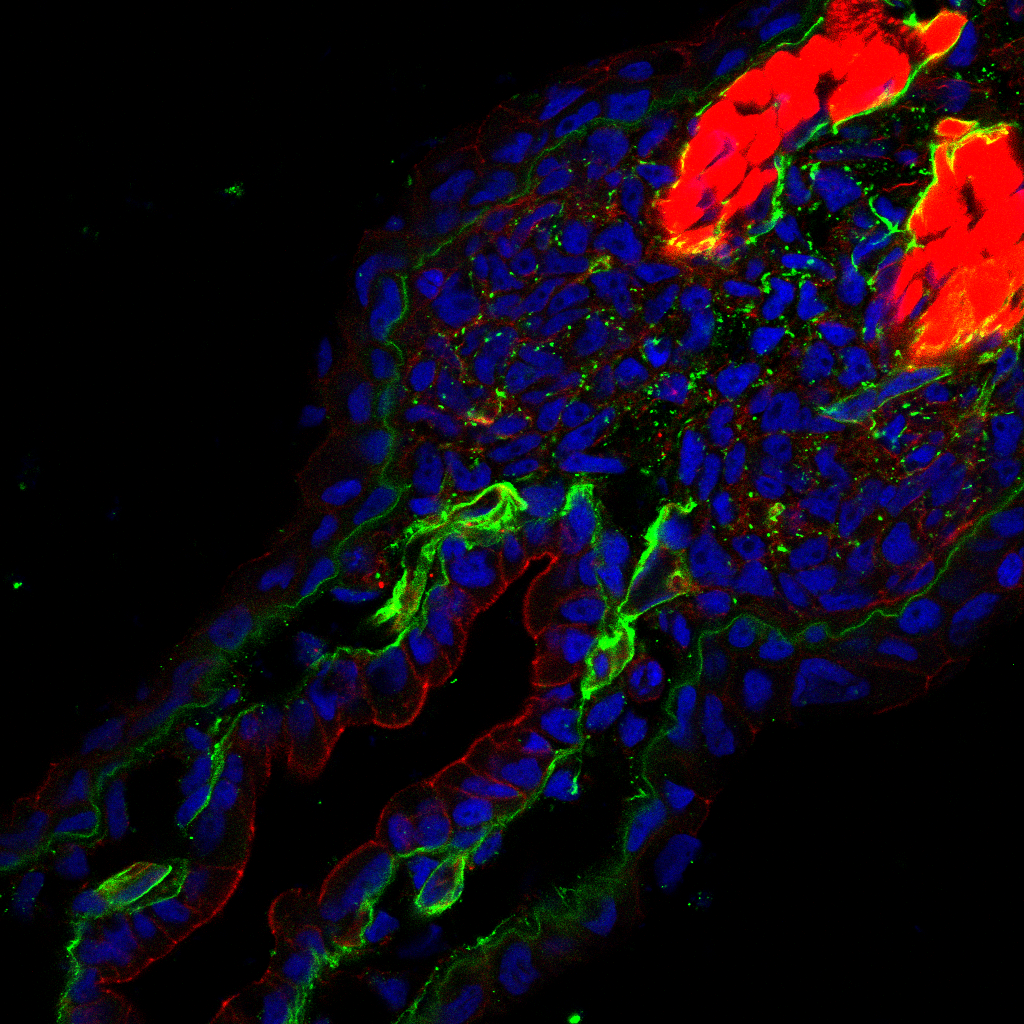
Limb Development in Anurans
The defining feature of tetrapods is possession of two sets of paired appendages. This group of terrestrial vertebrates contains the amphibians, reptiles, birds, and mammals. Even cetaceans with only two appendages, as well as legless lizards and snakes, evolved from four-limbed ancestors. These shared structures have attracted considerable attention from both developmental and evolutionary biologists. As a result, the study of the limb has provided a wealth of information about cell-cell communication, developmental patterning, and cell differentiation. Further, use of various model systems has demonstrated that early patterning of the limb is widely conserved across amniotes, a subset of tetrapods that include reptiles, birds, and mammals. Later variations in that developmental pattern generate the diversity of limb structure and form seen in these animals.
The frog Xenopus laevisis a widely used model system in developmental biology. It has a biphasic life history, meaning it has a transient larval stage before metamorphosing into its adult form. This life history is ancestral for extant amphibians, but is not thought to represent the ancestral state for tetrapods. A major consequence of this biphasic life history is that limb buds develop just before metamorphosis. Formation of the limb long after embryogenesis presents a striking contrast to early, embryonic, formation of the amniote limb. Taken together, these observations reveal a major gap in our knowledge. It remains unclear how anurans (frogs and toads) are capable of building their limbs during late tadpole stages. Furthermore, which tissues generate the limb in amphibians and how initiation is controlled genetically still has yet to be resolved.
It is pivotal to understand how this group of tetrapods are able to generate appendages during developmental stages where embryonic tissue is no longer present. Resolving this question will shed light on the cellular and genetic mechanisms that generate embryonic tissue in post-embryonic organisms. These findings will have implications for both evolutionary as well as regenerative biology. We use various molecular, genetic, and embryological techniques to understand where frog limbs come from and how they are generated.
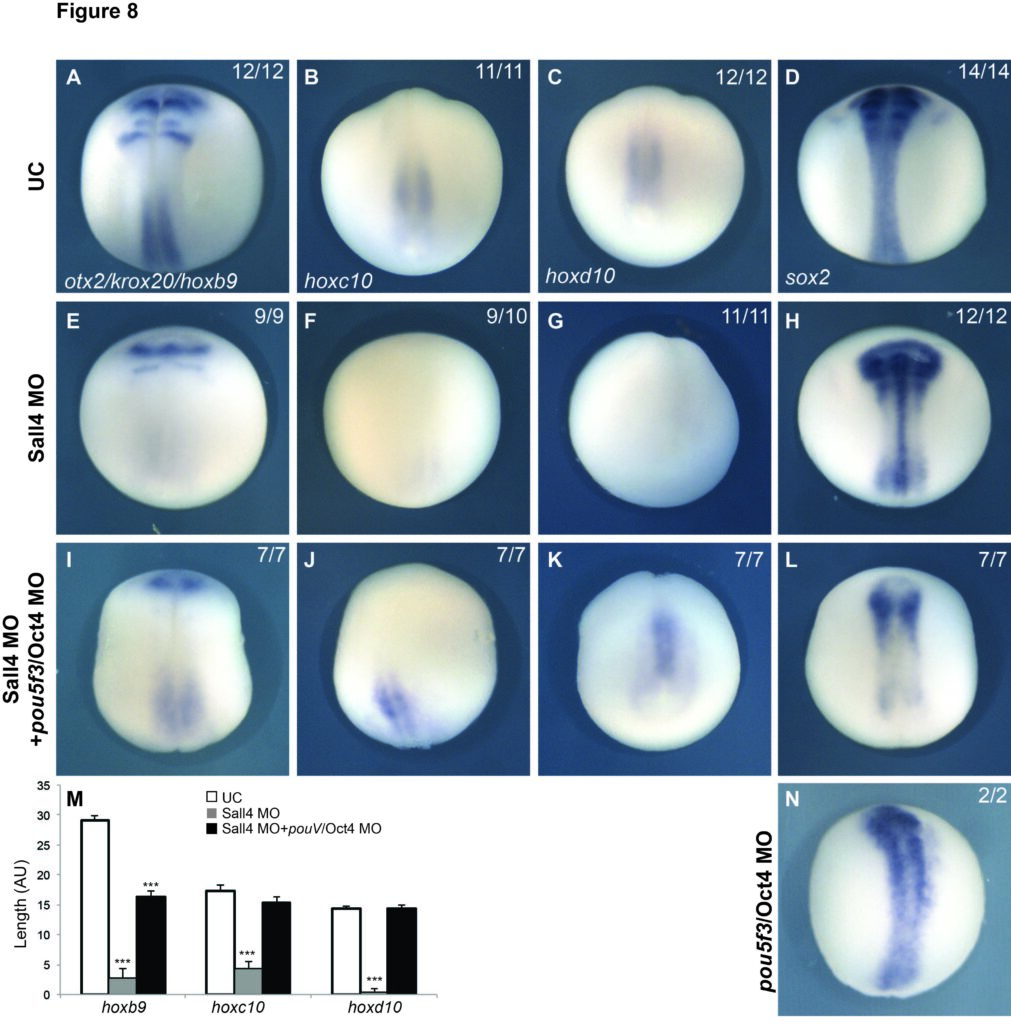
Anterior Posterior Patterning of the Neural Plate
Whether you are frog or a human, a central nervous system (CNS) that is not centrally located would not function properly. Therefore, it is incredibly important that your brain and spinal cord are positioned in the correct place in your body. Normal embryonic development ensures that the cells that make your brain are always in your head and those of your spinal cord are always protected within the vertebrae. Serious birth defects such as anencephaly and spina bifida occur when this process is disrupted. Yet, despite its importance, we know surprisingly little about how the CNS is patterned at the cellular level.
Previous work has discovered the protein Wnt to act as a critical signaling molecule that imparts the pattern of placing the brain in the head and the spinal cord in the backbone behind the brain. It does this by inducing the CNS cells in the posterior to adopt a spinal cord fate. While this work was important in understanding the initial process of pattern formation, it does not explain how those cells interpret the information provided by Wnt proteins and differentiate accordingly. Basically, how do the cells “know” to adopt a spinal cord identity when they receive this Wnt signal? The leading hypothesis to explain this process is that key pieces of DNA in the genome mediate the transfer of information from the Wnt signal to the precursor cells, resulting spinal cord fate.
We use standard molecular techniques alongside the widely available CRISPR/Cas9 system to understand how information encoded in secreted molecules is translated into positional identity. We use these methods to build a gene-regulatory network (GRN) to illustrate how patterning genes are regulated and interact to ensure reliable and repeatable development of the central nervous system.
Effects of the Industrial Chemical PFOS on Embryonic Development
Amphibians are exquisitely sensitive to contaminants in the environment due to their absorbent skin and lifestyle of living in both aquatic and terrestrial environments. Accordingly, they make an excellent model to assay the effects of potentially harmful toxins used in industrial purposes.
In collaboration with the Luth lab, we are investigating the any developmental defects that occur due to exposure of PFOA and PFOS.